|
 |
Scientific
Subjects
The computer
user syndrome |
Journal of the American Optometric Association,
November 1987, by Alan H. Grant
Why do virtually all computer operators suffer
to some degree from headaches, asthenopia, fatigue, various types
of head-neck-shoulder distress, as well as hand-wrist discomfort?
Simply because most existing computer hardware designs have not
adequately taken into account the way human beings would be functionally
most comfortable in performing ocular and orthopedic near-point
tasks. Rather, the individual computer hardware components developed
first and have been juxtaposed in the most convenient manner, and
we the users are in effect, the "squares pegs" who have had to accommodate
ourself to fit the "round holes."
The internal adjustment options and the external
add-ons to the existing systems, (i.e., Polaroid or mesh overlay
screens, various image/background colors, differential screen resolutions,
luminance/contrast controls, ergonomic chairs and work stands) all
contribute incrementally to the user comfort and efficiency.1,2
We are seriously disadvantaged however, because we are starting
from the circumstance of poorly designed and integrated computer
components.
To create a more ideal working environment,
a new schema has been postulated which integrates the ocular neurology
and orthopedic biomechanics.
Ocular neurology
The traditional concept of the Near Triad comprising
the three linked, neurological functions of accommodation, convergence,
and miosis, should be expanded to include two additional phenomenon:
depression-of-gaze and excyclotorsion. For want of a better entitlement,
we have named this multiple happening the Near Quintad Function.3
While the depression-of-gaze component can be volitionally overridden
so as to perform near-work visual tasks which are both close and
"high" in the visual field, it is this very act which provokes ocular
distress. It has been documented that excyclorotation increased
with convergence,4 and that this phenomenon associated
with convergence is increased with elevation-of-gaze, and is diminished
with depression-of-gaze.5
This would appear to be a violation of Listing's
Law, which describes monocular movements only about X and Y axes,
and which makes no provision for rotational movement about the Z
axes. Allen and Carter so concluded this, and suggested that excyclotorsion
be included as part of the near reflex.6
These cyclofusional movements have been classified
as psycho-optical responses which are first initiated by attention
to the target appearance, and then responded to by reflex. To the
extent that there is less excyclotorsion, there will be less cyclofusional
movement of the eye to restore and maintain the visual "vertical"
in the interest of sustaining single binocular vision, and the by-product
of this would be less expenditure of energy (and presumably less
fatigue) (Table 1).
Table 1
Disinclination of the vertical retinal
meridian because of exyclorotation associated with elevation-of-gaze
and depression-of-gaze* |
|
Elevation of Gaze |
Depression of Gaze |
|
20 degrees |
0 degrees |
20 degrees |
40 degrees |
Convergence |
6 degrees |
2.00 |
1.45 |
1.00 |
1.30 |
12 degrees |
3.40 |
2.55 |
2.00 |
1.30 |
25 degrees |
8.00 |
5.52 |
4.00 |
2.00 |
* (Excerpted from Modified
Landolt Table, and reproduced by permission from von Noorden,
Gunter K. Burian-von Noorden's binocular vision and ocular
motility, 3rd ed. St. Louis: CV Mosby: 1985.) |
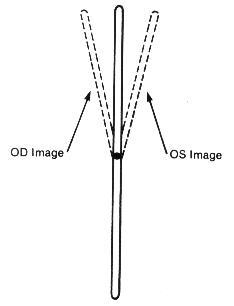 |
Figure 1
Monocular vertical target excyclorotation
with elevation-of-gaze. |
Experimentally it has been demonstrated that
relatively small target variations from parallelism of 5-8% will
create the subjective experience of blurring, disruption, and diplopia.7
It can often be demonstrated monocularly that there is an inward
rotation of the upper end of a vertical target line as the gaze
is shifted from a depressed position, through the primary and into
an elevated position. In this circumstance there would be an excyclorotated
retinal image, and sequentially a compensatory extraocular muscle
excyclotorsion (Figure 1). This effect can also be noted monocularly
with a tipping backward of the upper end of a vertical target line
(Figure 2).
Similarly, studies of the incomitance of heterophorias
relating to elevation-of-gaze and depression-of-gaze have indicated
that the predominant phoric variation is that of a "V" pattern (in
which there is more exophoria associated with elevation-of-gaze,
and less exophoria associated with depression-of-gaze). This effect
was more pronounced with near-point fixation of 33cm, than at a
3m testing distance. The "V" pattern incidence and extent increased
with larger gaze elevation/depression angles (from 20-40o).8
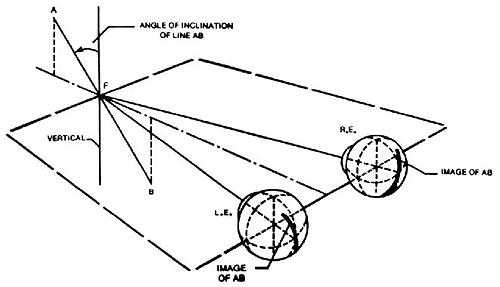 |
Figure 2
Excyclorotated retinal images with viewing
a superior inclined-away vertical target line. (Ogle KN. Researches
in binocular vision. Philadelphia/London: WB Saunders; 1950.) |
Over a long time-span, evolutionary changes
occurred permitting human forebears, out of necessity, to view distant
objects clearly and with some sense of depth. Duke-Elder comments
that "biologically the eyes were adapted for relatively simple purposes
- to look for enemies and for food."9 Large overlapping
visual areas producing binocularity became prerequisite to predatory
skills.10 To sustain the advantages of single binocular
vision, cyclofusional ocular movements became a necessity " . .
. in order that vertical objects such as walls, trees and so forth
may maintain their orientation as one raises or lowers the eyes
to look at various parts. . . ."7
If a valid measure of our human uniqueness is
that of being tool-makers and tool-users, then it logically follows
that we would have to be looking clearly at the end of our forelimb
appendages to accomplish the task at hand (figuratively and literally).
The side-benefit of the binocularity would have been a greater stereopsis
sense at near-point because of the significant lateral displacement
of the eyes in the skull (4-7cm), and the increased amount of convergence
being used.
While on all fours in an early quadripedal age,
the linear distance from the plane of our eyes to the ground between
our unflexed forelimbs was only incrementally greater than a normal
reading position distance for modern man. Whether we were still
herbivores breaking apart nuts, or had become omnivores and were
now also picking apart the carcass of a kill, these tasks would
have been easier looking downward.
Once the tool manufacturing was completed, utilization
still required point-of-use visual clarity (the keyboard), and point-of-resultant-tool-usage
clarity (the screen), and while prior experience might partially
and temporarily supplant the visual-touch mechanism, memory alone
is unequal to ongoing, efficient task performance. We simply have
to be looking at the things we do.
In our current bipedal mode, it is obvious that
we would expend less energy in tool manipulation if our forelimbs
were in that position that least conflicts with gravity (a rather
practical method of conserving physical strength by not bearing
the weight of our forelimbs in an unsupported and elevated attitude).
The result is a happy synchronicity of the most comfortable tool-using
posture with depression-of-gaze.
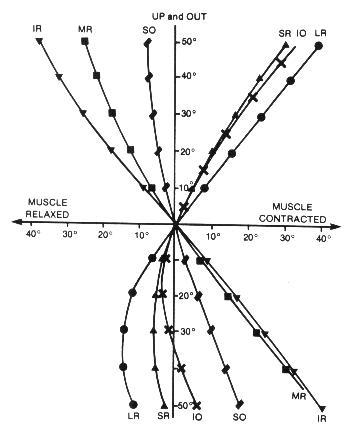 |
Figure 3
Depression and adduction: Four
muscle synergism plus superior rectus action. (Solomons H. Kinematics
of the extraocular muscles. Ophthal Opt 1977; 17:180.) |
Is the proposed Near Quintad Function neurological,
or is it simply that during convergence, extraocular muscle synergism
provides greater action by the combination of those muscles resulting
in a depressed position of gaze and consequently less excyclotorsion?
It has been established that the closeness of the insertion of the
extraocular muscle to the limbus, and the greatness of its muscle
mass will determine its relative activity during contraction. The
medial rectus ranks first in both categories, and the inferior rectus
has the next closest insertion to the limbus, and the third widest
tendon diameter (insertion spiral of Tilleau).11 Further
from Duke-Elder, "Thus convergence, so constantly required for near
work, with the exception of its presence to slight degrees in the
higher apes, is a new function unique to man and is frequently one
of the first to break down in the conditions of strain."9
Many researchers have studied and detailed differing conclusions
regarding binocular extraocular muscle function, but the consensus
is that:
Any position of gaze is the sum total of cooperation
of all the extraocular muscles, and that any schematic model of
individual muscle action would have to be considered hypothetical.
There are different sets of muscle planes and axes of rotation for
each position of gaze because the insertions of, and the states
of contraction of the extraocular muscles are changed for each position
of gaze.12
And what is contradictory is that:
"In the primary position, the axis of the horizontal
muscle pair is almost precisely vertical.... In extreme adduction,
however, the axis inclines about 8o... with the result
that there are small components of depression and excycloduction."12
Solomons has shown the variations of extraocular
muscle action for various positions of gaze and comments (with regard
to primary muscle actions) that "... In the horizontal meridian,
the lateral rectus abducts and the medial rectus adducts; however
they both elevate the eye very slightly, with the lateral rectus
being slightly more effective." However, while detailing secondary
and tertiary actions of the extraocular muscles it becomes apparent
that each individual muscle contributes differential contractive
and torsive efforts to the resultant ocular position. With adduction,
only the inferior rectus and superior oblique exert depressive effort.
With depression, there is now synergistic effort by four muscles
to effect/sustain this position: medial rectus, inferior rectus,
superior oblique, lateral rectus. With depression/adduction, there
are five muscles which contribute (the superior rectus now adds
to adduction).
Further, according to Solomons, " ... When the
eye looks 'down and in,' the inferior rectus is the strongest acting
muscle, aided almost equally by the medial rectus, and to a lesser
extent by the superior oblique. For movements greater than about
35o the inferior oblique also becomes involved"13
(Figure 3).
There have been animal studies in which the
extraocular muscles in monkeys have been severed and the insertions
transposed (medial rectus/lateral rectus), and where the superior
oblique muscle insertion has been substituted for a rectus muscle
insertion. Postsurgically within days, the researchers found the
ocular motility of the eye to have returned to its preoperative
condition.14 This suggests other nerve pathways of stimulation-response
which influence ocular movements. Despite these observational differences,
what is implied is that accommodation/convergence/miosis are in
some manner linked with depression-of-gaze and excyclotorsion.
According to Birnbaum, "Although efficient near-point
function requires precise matching between accommodation and convergence,
a tendency for convergence to localize closer than accommodation
is intrinsic to the near-point demands imposed by our culture. The
high level of psychological stress pervasive in our society is an
additional factor generating sympathetic activation and overconvergence.15
The convergence needed to perform near-work
tasks at a 40cm distance, with an assumed average interpupillary
distance of 63mm, results in angular deviation from parallelism
of 12o. Shortening the working distance to 20cm doubles
the angular deviation to 24o. Typically though, accommodation
does lag behind convergence resulting in an average of 4.2 diopters
of accommodation for each prism diopter of convergence (as opposed
to an ideal geometric match of 6 diopters: 1 prism diopter). What
then is the mechanism to ensure near-point target clarity?
With ocular synkinesis (The Near Triad), although
there is intermediation between the component functions, it is the
disparate images that initiate vergences first, which then affects
the state of accommodation (in seeking a clear image at the new
"verged" position), which then causes pupillary miosis (resulting
in an increased depth of focus), which then lessens some of the
accommodative effort required for a clear target image. Further,
"The pupil is known to constrict with sleepiness, boredom, or fatigue
and, if constricted, would increase the depth of focus, reducing
the requirement for accommodation."16
Therefore, to whatever near-point the eyes are
accommodated for, we are overconverged upon a yet closer near-point,
and there would be increased excylotorsion.
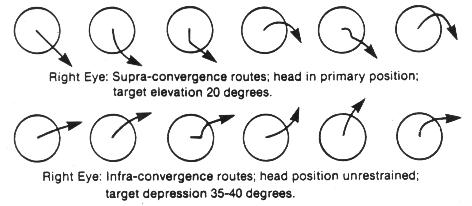 |
Figure 4
Primary, supra, infra convergence
routes |
Borish has stated: " . . . The eye is seldom
turned 10-12o, as head movements complete the needed
angular turn for most fixations. In theory the movement possible
is only 1/8 or 1/9 of the total."17 Von Noorden supports
this view that measurements of eye positions exceeding 15o
from the primary have little significance in our functional visual
lives, and cites other research that horizontal ocular excursions
were minimal (6-8o) for most visual functions. Generally,
ocular movements preceded head movements, and the head movements
were greater with increased difficulty of the near-work task.5
The simplest way to demonstrate the depression-of-gaze
effect is to conduct a push-up-convergence test by having the subject
in a standing or seated position with a primary head position, and
then introducing a small, finite target brought directly inward
along the midline of the primary horizontal plane of the two eyes.
The subject's eyes will at some point during converging, be in a
depressed gaze position, and will achieve this position by various
routes. Each eye may also travel a different path while converging.
If this finite target is introduced from above
the primary horizontal, what is observed is a converging-inward,
sometimes upward-arcuate-downward ocular movement, which always
ends in a lowered ocular position. When the finite target is introduced
from below the primary horizontal (and permitting the subject to
drop the head forward and downward into whatever position is most
natural), what is observed is principally a converging movement
with a slight upward component that can occur at the beginning,
midpoint, or toward the end of the sequence (Figure 4).
This author has observed and detailed push-up-convergence
responses of 100 individuals (all ages, both sexes, all refractive
errors) for three positions. The finite target was moved inward
along the midline from 60cm to 20cm. In the primary and supra positions,
the head position was restrained in an upright posture because otherwise,
the subject would have tended to elevate the chin and tip the head
backward slightly (capitas extension) as the target approached,
and this would have produced a false appearance of convergence without
a depression-of-gaze component.
Capitas extension is noted in pre-presbyopes
and is exaggerated in over 40 operators who gradually need supplemental
presbyopic corrections to see clearly the screen/keyboard/copy-viewing
area, and because supraduction ability is also lessening. If screens
were placed at least 35-40o below the primary horizontal
plane, and angled backward 45o to the vertical, then
the wearing of conventional multifocal spectacle corrections would
eliminate the need for this orthopedic imposture.
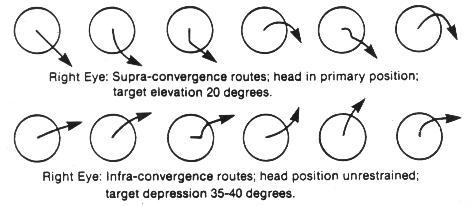 |
Figure 5
Capital extension and capitas
flexion. |
In virtually all the primary-convergence observations,
a depression-of-gaze component was noted. With infra-testing, the
head position was left free so that the target-viewing was the combination
of both head flexion and ocular depression-of-gaze. What this illustrates
is that, either through volition or involition (or both), the eyes
will migrate to a depressed position-of-gaze during the adductive
process. Further, virtually every subject notices less subjective
comfort in trying to converge upon an incoming target from a straight
ahead or elevated position. The greatest subjective degree of comfort
occurs when convergence and depression-of-gaze are combined. That
supraduction function, diminishing from an average capability of
40o (patients age 5-14) to 16o (patients age
85-94),18 supports the depression-of-gaze/maximum comfort
thesis.
Occasionally with conventional computer usage,
a capitas-flexion position is noted which creates a false elevation-of-gaze.
It is probable that this operator has opted to bear with whatever
distress the increased supraduction and excyclotorsion will produce,
and to avoid capitas-extension postural difficulties (Figure 5).
IBM Human Factors Center states that "a comfortable
line of sight is 10o or more below the horizontal." (My
observation is that this is only partially correct.) "Hence, the
display should be located so that the normal line of vision falls
in the upper half of the display."19 (My observation
is that this is stressful to the user.) The National Research Council
cites "a preferred downward slope of the line of sight from 15-30o."16
(My observation is that this is still inadequate.) This compromise
of good ocular function was probably accepted as means of minimizing
reflected glare that would have accompanied the sufficient lowering
and angling backward of the screen.
With existing computer usage, we are utilizing
relatively large saccades (30-45o) to continually reposition
the screen/keyboard/copy-viewing-area targets onto the fovea. With
large angular-difference refixations, transient dysmetria (under-shooting
the subsequent fixation point) is not uncommon.18
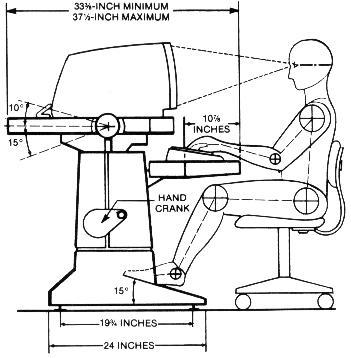 |
Figure 6
Ergonomic work station
(Rubbermaid Corp). |
There is unavoidable perceptual disorientation
of visual space created by torsional imbalances when the eyes are
in a tertiary position.10 This condition is persistive,
because astigmias corrected with a spectacle Rx or hydrophilic contact
lenses are based upon examination techniques derived while the head
and eyes are in a primary position. Rarely do we encounter astigmias
which are totally corneal and with no residual component, so that
the refractive error is fully corrected with a rigid contact lens
prosthetic (in this instance, torsional imbalances would not be
exacerbated by working in a skewed position). Therefore copy-viewing
areas placed laterally and inferiorly to typewriters and computers
are poor. Ideally the copy-viewing area should be along the visual
mid-line and at least 35-40o below the primary horizontal
plane (Figure 6).
Although field-of-fixation (an area within which
fixation can be accomplished by moving only the eye and not the
head) is clinically demonstrated to be relatively large (40-50o),
it is less binocularly than monocularly, and its amplitude diminishes
with age. The presence of a stiff neck reveals the subjective difficulty
in executing ocular, sans head movements.5
The pursuit system which matches eye velocity
to target velocity, in viewing a 7" x 9" screen from an 18" working
distance, will have to encompass an approximate angular range of
30+o vertically and 40+o horizontally. Following
the advancing cursor as data is inputted, appears to be well within
that speed that would detract from the comprehension of the operator.
Vertical scrolling speed however, appears to exceed comprehension
levels, and it is uncertain whether vertical scanning capability
is being detracted from by successively appearing targets.
References
- American National Standards
for Human Factors Engineering of Visual Display Terminal Workstations:
Human Factors Society; POB 1369, Santa Monica, California, 90406;
1985.
- Mills CB, Weldon LJ. Reading
text from computer screens. College Park, MD: Center for Automation
Research, Human-Computer Interaction Laboratory, University of
Maryland, 1985.
- Grant A, Grant L. CRT users,
CL candidates. Contact Lens Spectrum 1986; 1(4):50.
- Alpern M. Movements of the
eyes, vol 3. New York: Academic Press, 1962.
- Von Noorden GK. Binocular
vision and ocular motility: theory and management of strabismus,
3rd ed. St. Louis: CV Mosby, 1985: 64, 81-2.
- Allen MJ, Carter JH. The
torsion component of the near reflex. Am J Optom Arch Am Acad
Optom 1967; 44:349.
- Ogle KN. Researches in binocular
vision. Philadelphia/London: WB Saunders, 1950: 101,118.
- Stuart JA, Burian HM. Changes
in horizontal heterophoria: with elevation and depression of gaze.
Am J Ophthalmol 1962; 53:274.
- Duke-Elder S, ed. The neurology
of vision motor and optical anomalies. In: Textbook of ophthalmology,
vol 4. London: Henry Kimpton, 1950: 4466.
- Bennett AG, Rabbetts RB.
Clinical visual optics; requirements for binocular vision. London:
Butterworths, 1984: 148, 151.
- Wolff E. The anatomy of the
eye and orbit, 3rd ed. Philadelphia: Blakistan, 1949: 209.
- Boeder P. Cooperation of
extraocular muscles. Am J Ophthalmol 1961; 51:469.
- Solomons H. Kinematics of
the extraocular muscles. Ophthal Opt 1977; 17: Issues 1/8, 1/22,
2/5, 2/19, 3/5.
- Marina A. Die theorie uber
den mechanismus der assozuerten konvergenz. Dtsch Z Nervenheilkd
1912; 44:138.
- Birnbaum MH. Symposium on
near-point visual stress. Am J Opt Physiol Opt 1985; 62:361.
- Panel on impact of video
viewing on vision of workers. Video displays, work and vision.
Washington, DC: National Academy Press, 1983: 141, 167.
- Borish I. Clinical refraction,
vol 1, 3rd ed. Chicago: Professional Press, 1970: 193.
- Miller NR. Clinical neuro-ophthalmology,
4th ed. Baltimore/London: Williams & Wilkins, 638, 647.
- Human factors of work stations
with visual displays. Human Factors Center; IBM Corporation; San
Jose, California 95193;1984:45.
Return
to Top of Page
Back
Reproduced from
the November 1987 issue of the Journal of the American Optometric
Association
|